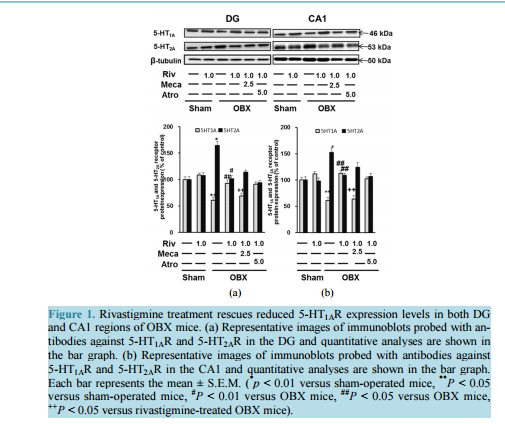
Rivastigmine Restores 5-HT1A Receptor Levels in the Hippocampus of Olfactory Bulbectomized Mice Muhammad Rashedul Islam, Shigeki Moriguchi, Hideaki Tagashira, Kohji Fukunaga Department of Pharmacology, Graduate School of Pharmaceutical Sciences, Tohoku University, Sendai, Japan Email: kfukunaga@m.tohoku.ac.jp Received 10 July 2014; revised 20 August 2014; accepted 29 August 2014 Copyright © 2014 by authors and Scientific Research Publishing Inc. This work is licensed under the Creative Commons Attribution International License (CC BY). http://creativecommons.org/licenses/by/4.0/ Abstract Rivastigmine, a dual acetylcholinesterase and butyrylcholinesterase inhibitor, is used for symptomatic treatment of patients with mild to moderately severe dementia in Alzheimer’s disease (AD) patients. In the present study, we found that 5-HT1A receptor (5-HT1AR) is downregulated, whereas 5-HT2A receptor (5-HT2AR) is upregulated in the hippocampal dentate gyrus (DG) and CA1 region by olfactory bulbectomy (OBX) in mice. Furthermore, chronic treatment with rivastigmine (1.0 mg/kg) for 2 weeks starting 2 weeks after OBX operation restored the decreased 5-HT1AR and the increased 5-HT2AR levels. To determine whether cholinergic receptor stimulation by rivastigmine is involved in the rivastigmine-induced regulation of 5-HTR levels, we treated the mice with mecamylamine (2.5 mg/kg), or atropine (5.0 mg/kg) with rivastigmine (1.0 mg/kg) once a day for 2 weeks. Notably, the rivastigmine-induced 5-HT1AR upregulation was eliminated by mecamylamine but not by atropine treatments. On the other hand, the restored 5-HT2AR level by rivastigmine was not affected by either mecamylamine or atropine. Treatment with 8-OH-DPAT, a selective 5-HT1AR agonist improved the decreased 5-HT1AR and the increased 5-HT2AR levels in OBX mice. On the other hand, treatment with TCB-2, a potent 5-HT2AR agonist had no effects on the 5-HT1AR and 5-HT2AR dysregulation in OBX mice. Taken together, nicotinic acetylcholine receptor (nAChR) stimulation mediates rivastigmine-induced upregulation of 5-HT1AR. Therefore, we speculate that the increased ACh levels by rivastigmine can stimulate nAChR located on serotonergic nerve terminals and stimulate 5-HT1AR by the enhanced 5-HT release in the hippocampus. The 5-HT1AR stimulation likely mediates the improvement of 5-HT1AR levels as auto-receptor in OBX hippocampus. Keywords Rivastigmine, 5-HT1A Receptor, 5-HT2A Receptor, Nicotinic Acetylcholine Receptor, Olfactory Bulbectomized Mice M. R. Islam et al. 129 1. Introduction The hippocampal functions are delicately modulated by cholinergic and serotonergic inputs from basal forebrain and the raphe nuclei, respectively. For example, nicotinic acetylcholine (nACh) receptor on presynaptic terminal regulates memory and attention function in the hippocampus [1]. Likewise, 5-HT1AR regulates mood-related behaviors through somatodendritic inhibitory receptors pre-synaptically in the hippocampus [2]. 5-HT1AR is located post-synaptically in pyramidal and granular neurons of the hippocampus [3]. 5-HT2AR is also expressed in the pyramidal cell layers of CA1 and granule cells of dentate gyrus (DG) in the hippocampus of Sprague-Dawley rats [4], which has an important role in regulating network activity and neural oscillation in the hippocampal region [5]. In addition, 5-HT transporter (5-HTT) is localized to serotonergic neurons of the hippocampus, especially in the axons and nerve terminals [6] [7]. The presynaptically located 5-HTT is crucial for the regulation of 5-HT transmission because it controls the 5-HT availability at the site of postsynaptic receptors by high affinity reuptake of 5-HT in the synaptic cleft [8]. Olfactory bulbectomy (OBX) in rodents has been used as an Alzheimer disease (AD) model, because OBX induces various behavioral and biochemical phenotypes of AD such as increases in locomotor activity [9], aggressiveness [10] and cognitive defects [11]. Moreover, OBX in mice induces an elevation of amyloid beta (Aβ) in the brain [12]. We also reported neurodegeneration of cholinergic neurons in the medial septum [13] and impaired neurogenesis in the hippocampal DG [14]. Interestingly, OBX surgery degenerates 5-HT fibers innervating to the hippocampus, thereby reducing 5-HT synthesis and 5-HT receptor expression in rats. Therefore, this model is suitable for studying the cognitive deficits accompanied depressive symptoms [15] [16]. Likewise, post mortem examinations of brains reveal significant decline in both 5-HT and ACh levels as well as decreased hippocampal 5-HT1AR expression [17]-[19]. Rivastigmine is a second-generation, carbamate-based reversible non-competitive inhibitor of both acetylcholinesterase (AChE) and butyrylcholinesterase (BuChE) used to treat mild to moderate dementia in patients [20]. Recent studies report that AD patients administered rivastigmine show reversals in depression, as evaluated by the Hamilton Depression Scale [21]. Rivastigmine has been approved by the Food and Drug Administration (FDA) to enhance or maintain cognition in AD patients [22]. A current 6-month open-label observational study reported that treatment with a rivastigmine patch alone decreased the frequency and severity of major depression episodes (MDEs) in 50 patients with mild AD [23].We recently documented that rivastigmine improves deficits in cognition and memory-related behaviors in OBX mice [24]. In addition, we documented that rivastigmineinduced hippocampal neurogenesis and improvement of depressive-like behaviors in OBX mice is mediated by 5-HT1AR stimulation [25]. Since high dose (0.5 mg/kg, s.c.) of nicotine stimulates 5-HT release in the hippocampus and elicits anxiogenic effects in rats [26], rivastigmine-enhanced ACh levels likely stimulate 5-HT release in the hippocampus. Although how rivastigmine stimulate 5-HT release in the hippocampus remains unclear, it is important to investigate the physiological relevance of increased serotonergic neural activity. Therefore, to extend our earlier observations, we examined whether 5-HT1AR activation by rivastigmine regulates 5-HT1AR and 5-HT2AR levels in OBX hippocampus. 2. Materials and Methods 2.1. Animals 8 weeks-old male DDY mice weighing 23 to 26 g were obtained from Nippon SLC (Hamamatsu, Japan). All animals were group housed in our animal facility in propylene cages at a constant temperature (23˚C ± 1˚C) and humidity (55% ± 5%) with a reversed 12:12 light/dark cycle (lights off at 21.00 p.m.) and had free access to food and water. Animal Experimentation Committee on Animal Experiments at Tohoku University approved all experimental animal procedures. All efforts were made to reduce animal suffering and minimize the total number of animals used. 2.2. Operation and Treatment Diagram After one week of acclimatization period, OBX mice were prepared as described [11] [24]. Briefly, mice anesthetized with sodium pentobarbital (50 mg/kg i.p. Dainippon, Osaka, Japan) were placed in a stereotaxic instrument (KOPF, Tujunga, California, USA). After exposure of the skull, 1-mm diameter holes were drilled on ei- M. R. Islam et al. 130 ther side of the olfactory bulbs, which were then bilaterally, aspirated using a suction pump. Care was taken not to damage the frontal cortex. Holes were filled with a homeostatic sponge (Spongel, Astellas Pharma Inc., Tokyo, Japan) to avoid further bleeding, and the skin was closed with sutures. Sham-operated animals underwent the same procedure without excision and aspiration of olfactory bulbs. After surgery, all animals were allowed to recover in a post-operative cage (maintained at 23˚C) for 3 hr. After this period, mice were returned to their home cage. After the 2 weeks of recovery and appearance of depression-like behaviors [27], rivastigmine (Exelon, Novartis Pharma, AG, Basel, Switzerland) was administered (1.0 mg/kg; s.c) 30 min after a nAChR antagonist, mecamylamine (2.5 mg/kg, s.c) or a mAChR antagonist, atropine (5.0 mg/kg, i.p.) administration once daily for 14 days. Similarly, a selective 5-HT1AR agonist, (7)-8-hydroxy-2-(di-n-propylamino) tetralin(8-OH-DPAT) (1.0 mg/kg; s.c.; Tocris Bioscience, Bristol, United Kingdom); and a selective 5-HT2AR agonist, (4-bromo-3,6-dimethoxybenzocyclobuten-1-yl) methylamine (TCB-2) (1.0 mg/kg; i.p.; Tocris Bioscience) was administered once daily for 14 days. The doses and administration protocols of drugs used in this study were selected based on findings from the literature employing these drugs in animal behavioral models [25] [28] [29]. 24 hrs after the last dose, all mice were sacrificed for western blot analysis and the lesions were verified histologically. 2.3. Experimental Animal Groups Mice were first separated into the following six groups (n = 7): I: sham; II: 1.0 mg/kg rivastigmine-treated sham; III: OBX; IV: 1.0 mg/kg rivastigmine-treated OBX; V: 2.5 mg/kg mecamylamine co-administered with 1.0 mg/kg rivastigmine in OBX, and VI: 5.0 mg/kg atropine co-administered with 1.0 mg/kg rivastigmine in OBX. In experiments assessing receptor agonists, mice were again assigned to four groups (n = 7). Groups included: I: sham; II: OBX; III: 1.0 mg/kg 8-OH-DPAT treated OBX, and IV: 1.0 mg/kg TCB-2 treated OBX. 2.4. Immunoblot Analysis Immunoblot analyses were performed as described previously [14]. Samples were dissected out from experimental mice brain and were homogenized in 70 μl buffer containing 50 mm Tris-HCl, pH 7.4, 0.5% Triton X- 100, 4 mm EGTA, 10 mm EDTA, 1 mm Na3VO4, 40 mm sodium pyrophosphate, 50 mm NaF, 100 nm calyculin A, 50 μg/ml leupeptin, 25 μg/ml pepstatin A, 50 μg/ml trypsin inhibitor and 1 mm dithiothreitol. Insoluble material was removed by a 10-min centrifugation (15,000 rpm). After determining supernatant protein concentration using Bradford’s solution, samples were heated at 100˚C for 3-min in Laemmli’s sample buffer. Now samples containing equivalent amounts of protein were subjected to SDS-polyacrylamide gel electrophoresis. Proteins were transferred to an Immobilon polyvinylidene difluoride membrane for 2-h at 70 V. After blocking with TTBS solution (50 mm Tris-HCl, pH 7.5, 150 mm NaCl and 0.1% Tween 20) containing 5% nonfat dry milk for 1-h at room temperature, membranes were incubated overnight at 4˚C with anti-5HT1A receptor antibody (1: 1000, ab85615, Abcam, Cambridge, MA, USA); anti-5HT2A receptor antibody (1:1000, ab16028, Abcam, Cambridge, MA, USA), anti-Serotonin Transporter (5-HTT) antibody (1:2000, AB9726, Cell signaling, Woburn, MA,USA) and anti-beta-tubulin (1:5000,Sigma-Aldrich, St. Louis, MO, USA). Bound antibodies were visualized using the enhanced chemiluminescence detection system (GE Healthcare, Chalfont St. Gilws, UK) and analyzed semiquantitatively using the National Institute of Health Image program (Bethesda, MD, USA). 2.5. Statistical Evaluation All data were expressed as means ±S.E.M. Comparison between two experimental groups was made using the unpaired student’s t test. Statistical significance for multiple comparisons between control and other groups uses Scheffe’s test by SPSS software (IBM). Statistical significance was considered P < 0.05. 3. Results 3.1. Involvement of Cholinergic Effect in Rivastigmine-Induced 5-HT1AR and 5-HT2AR To explore the influence of rivastigmine on the expression of 5-HT1AR and 5-HT2AR, western blotting was first performed in DG samples. As shown in Figure 1(a), in sham-operated mice, quantitative analyses revealed that rivastigmine treatment had no effect on expression of 5-HT1AR or 5-HT2AR in the DG. M. R. Islam et al. 131 (a) (b) Figure 1. Rivastigmine treatment rescues reduced 5-HT1AR expression levels in both DG and CA1 regions of OBX mice. (a) Representative images of immunoblots probed with antibodies against 5-HT1AR and 5-HT2AR in the DG and quantitative analyses are shown in the bar graph. (b) Representative images of immunoblots probed with antibodies against 5-HT1AR and 5-HT2AR in the CA1 and quantitative analyses are shown in the bar graph. Each bar represents the mean ± S.E.M. ( * p < 0.01 versus sham-operated mice, **P < 0.05 versus sham-operated mice, # P < 0.01 versus OBX mice, ##P < 0.05 versus OBX mice, ++P < 0.05 versus rivastigmine-treated OBX mice). On the other hand, we first observed decreased 5-HT1AR (64.67% ± 2.05% of sham, P < 0.05 vs. sham) and in contrast increased 5-HT2AR (164.12% ± 1.78% of sham, P < 0.01 vs. sham) expression both in the DG region (Figure 1(a)). Interestingly, chronic rivastigmine treatment improves the dysregulation of 5-HT1AR and 5-HT2AR (5-HT1AR: 96.16% ± 1.64% of sham, P < 0.05 vs. OBX; 5-HT2AR: 101.19% ± 2.26% of sham, P < 0.01vs. OBX). Notably, the improvement of 5-HT1AR levels by rivastigmine was inhibited by mecamylamine co-administration (65.72% ± 1.84% of sham, P < 0.05 vs. rivastigmine-treated OBX mice), while atropine administration had no effect (90.08% ± 3.51% of sham). On the other hand, restoration of 5-HT2AR levels by rivastigmine treatment was not blocked by mecamylamine and atropine. This suggests that nAChRs might be involved in rivastigmine-induced up-regulation of 5-HT1AR expressions in the DG of OBX mice. Similarly, the dysregulation of 5-HT1AR and 5-HT2AR was observed in the CA1 region and rivastigmine treatment improved the decreased 5-HT1AR (112.58% ± 1.37% of sham, P < 0.05 vs. OBX) and increased 5- HT2AR expression (108.70% ± 1.93% of sham, P < 0.05 vs. OBX) in OBX mice (Figure 1(b)). Likewise, mecamylamine antagonized the rivastigmine effect on 5-HT1AR (63.81% ± 2.42% of sham, P < 0.05 vs. rivastigmine-treated OBX mice), without alteration in 5-HT2AR levels. 3.2. Effect of 8-OH-DPAT and TCB-2 on 5-HT1AR and 5-HT2AR Protein Expression Since nAChR mediates regulation of 5-HT1AR expressions by rivastigmine, we speculated nAChR stimulates 5-HT release, thereby up-regulating 5-HT1AR in OBX mice. Therefore, we tested effects of 5-HTR agonists on the dysregulation of 5-HT1AR and 5-HT2AR expressions following OBX. After administration of 8-OH-DPAT or TCB-2, we measured 5-HT1AR and 5-HT2AR expressions in both DG and CA1 regions. As expected, 5-HT1AR agonist, 8-OH-DPAT treatments significantly improved the decreased 5-HT1AR levels (DG: 134.67% ± 2.42% of sham, P < 0.01 vs. OBX; CA1: 155.93% ± 3.62% of sham, P < 0.01vs. OBX) (Figure 2(a), Figure 2(b)). In addition, the increased 5-HT2AR levels in OBX mice (DG: 143.41% ± 2.48% of sham, P < 0.05 vs. sham; CA1: 155.25% ± 2.99% of sham, P < 0.05 vs. sham) were restored in 8-OH-DPAT treatment groups of OBX mice (DG: 96.05% ± 3.50% of sham, P < 0.01 vs. OBX; CA1: 103.98% ± 1.70% of sham, P < 0.01 vs. OBX) (Figure 2(a), Figure 2(b)). Whereas, 5-HT2AR, agonist, TCB-2 treatment M. R. Islam et al. 132 (a) (b) Figure 2. 8-OH-DPAT elevates 5-HT1AR expression levels in both DG and CA1 of OBX mice. (a) Representative images of immunoblots probed with antibodies against 5-HT1AR and 5-HT2AR in the DG and quantitative analyses are shown in the bar graph. (b) Representative images of immunoblots probed with antibodies against 5-HT1AR and 5-HT2AR in the CA1 and quantitative analyses are shown in the bar graph.Each bar represents the mean ± S.E.M. ( ** P < 0.05 versus sham-operated mice, # P < 0.01 versus OBX mice). failed to improve 5-HT1AR and 5-HT2AR expression in the DG and CA1 of OBX (Figure 2(a), Figure (2b)). These observations suggest that activation of 5-HT1AR by 8-OH-DPAT restores both 5-HT1AR and 5-HT2AR expressions in the DG and CA1 of OBX mice. 3.3. Effect of Rivastigmine, 8-OH-DPAT and TCB-2 on 5-HTT Protein Expression Since 5-HT transporter (5-HTT) has crucial role in the regulation of 5-HT levels in synaptic regions, we next assessed the effect of rivastigmine on hippocampal 5-HTT protein levels in OBX mice. The significantly elevated 5-HTT levelsseen in the hippocampus of OBX mice (159.04% ± 2.98% of sham, P < 0.05 vs. sham) was restored by rivastigmine treatment (108.01% ± 4.27% of sham, P < 0.05 vs. OBX) (Figure 3(a)). Mecamylamine co-administration, but not atropine prevented the increased hippocampal 5-HTT protein levels (158.92% ± 5.25% of sham, P < 0.05vs. rivastigmine-treated OBX) (Figure 3(a)). Similarly, treatment with 8-OH-DPAT but not TCB-2 restored increased 5-HTT levels (147.09% ± 1.41% of sham, P < 0.05 vs. sham; 103.46% ± 3.03% of sham, P < 0.05 vs. OBX) (Figure 3(b)). Taken together, these findings confirm that rivastigmine improves the enhanced 5-HTT protein levels seen in the hippocampus of OBX mice and 5-HT1AR stimulation mediates the 5-HTT regulations by rivastigmine. 4. Discussion The novel findings in the present study are that the depression-like behaviors are closely associated with downregulation of 5-HT1AR and upregulation of 5-HT2AR in the hippocampal DG and CA1 after OBX and that rivastigmine restores the reduced 5-HT1AR in nAChR-dependent manner. Because mecamylamine co-administration, but not in atropine prevented the rivastigmine-induced 5-HT1AR expression in the hippocampus, the elevated 5-HT1AR expression was not due to a direct effect of rivastigmine on 5-HT1AR. We conclude that rivastigmineinduced ACh increase stimulates nAChR, which mediates the 5-HT1AR regulation by rivastigmine treatment. Furthermore, 5-HT1AR stimulation by release 5-HT accounts for regulation of both 5-HT1AR and 5-HT2AR in the hippocampus. Our results are supported by evidences that nAChRs are located on hippocampal serotonergic neurons in rats and are capable of modulating 5-HT release [30] [31] and that rivastigmine treatment increases the availability and binding of endogenous ACh at nicotinic receptors in methamphetamine-dependent individuals [32]. Furthermore, nicotine-induced increase in 5-HT release in rat hippocampal slices is antagonized by mecamyla- M. R. Islam et al. 133 (a) (b) Figure 3. Rivastigmine and 8-OH-DPAT restored elevated 5-HTT expression level in the hippocampus of OBX mice. (a) Representative images of immunoblots probed with antibodies against 5-HTT in the hippocampus and quantitative analyses are shown in the bar graph. (b) Representative images of immunoblots probed with antibodies against 5-HTT in the hippocampus and quantitative analyses are shown in the bar graph. Each bar represents the mean ± S.E.M. ( ** P < 0.05 versus sham-operated mice, ##P < 0.05 versus OBX mice, ++P < 0.05 versus rivastigmine-treated OBX mice). mine [33]. Rivastigmine significantly increased [ 3 H]-EPI binding (to measure non-α7 nAChRs) only, not [ 3 H]-MLA binding (to measure α7 nAChRs), in the hippocampus of Sprague-Dawley rats [34]. Previous behavioral findings also demonstrate that rivastigmine-improved neurological deficits induced by closed head injury in male Sabra rats wereprevented by the simultaneous injection of mecamylamine (2.5 mg/kg.), but not scopolamine (1.0 mg/kg), a mAChR antagonist [29]. Rivastigmine ameliorates neurological dysfunction and memory deficits after a chronic autoimmune encephalomyelitis model in female C57BL/6 mice induced by myelin oligodendrocyte glycoprotein. The effects are reversed by co-administration of mecamylamine [35]. Together with the previous findings, our results suggests that stimulation of nAChRs on 5-HT neurons through increased ACh causes 5-HT release, which activates 5-HT1AR in the hippocampus of rivastigmine-treated OBX mice. The finding on up-regulation of 5-HT1AR expressions in OBX mice following 8-OH-DPAT presented here fit well with 5-HT-mediated mechanism of up-regulation of 5-HT1AR. Furthermore, we confirmed that treatment with mecamylamine, atropine, 8-OH-DPAT or TCB-2 alone in sham or OBX mice had almost no marked effects on 5-HT1AR, 5-HT2AR and 5-HTT expressions (data not shown). Our data provide evidence that variation in hippocampal 5-HT1AR expressions can also affect the level of 5-HT2AR expressions. However, the precise causal pathway involved in the 5-HT1AR-mediated 5-HT2AR expressions did not define in the present study. To the best of our knowledge, this is the first study to demonstrate that rivastigmine restores 5-HT1AR and 5-HT2AR expression in the hippocampus of OBX mice. It is well documented that both 5-HT1AR and 5-HTT play an important role to maintain 5-HT homeostasis in the hippocampus. For example, the increased 5-HTT densities were observed in the hippocampus of OBX rats by autoradiography [36]. The elevation of the 5-HTT in the OBX rats is likely mediated by proliferation of the glia after the neuronal cells die [37]. Elevated 5-HTT protein levels in our study were decreased following rivastigmine or 8-OH-DPAT treatments. The improvement of 5-HTT levels by rivastigmine was prevented by mecamylamine co-administration. Taken together, nAChRs present in serotonergic neurons likely modulate the serotonergic transmission in the hippocampus as shown in Figure 4. In conclusion, nAChR is involved in rivastigmine-induced 5-HT1AR upregulation in the hippocampus of OBX mice. Stimulation of 5-HT1AR mediates improvement of impaired 5-HT1AR and 5-HT2AR in the hippocampus. To define the site of action of nAChR on the serotonergic neurons, further extensive studies are required. The novel site of action on 5-HT1AR induced by rivastigmine is attractive to explain the effects on the behavioral and psychological symptoms in Alzheimer disease patients. M. R. Islam et al. 134 Figure 4. Working model of rivastigmine in the modulation of hippocampal 5-HT neural activity. The elevation of ACh concentration by blocking of ChE activity by rivastigmine results in stimulation of nicotinic acetylcholine receptors located on serotonergic nerve terminals in the hippocampus, thereby increasing extracellular 5-HT levels. The stimulation of 5-HT1A receptors likely mediates upregulation of 5-HT1AR. 5-HT, serotonin; ACh, acetylcholine; ChE, cholinesterase; nAChR, nicotinic acetylcholine receptor; 5-HTT, serotonin transporter protein; 5-HT1AR and 5-HT2AR, serotonin receptor subtypes. Acknowledgements This work was supported in part by grants from the Ministry of Education, Culture, Sports, Science and Technology and the Ministry of Health and Welfare, Japan (KAKENHI 24102505, 25293124, KF) and Smoking Research Foundation (KF). Conflicts of Interest The authors declare that we have no conflicts of interest to disclose. References [1] Thomsen, M.S., Hansen, H.H., Timmerman, D.B. and Mikkelsen, J.D. (2010) Cognitive Improvement by Activation of Alpha 7 Nicotinic Acetylcholine Receptors: From Animal Models to Human Pathophysiology. Current Pharmaceutical Design, 16, 323-343. http://dx.doi.org/10.2174/138161210790170094 [2] Richardson-Jones, J.W., Craige, C.P., Guiard, B.P., Stephen, A., Metzqer, K.L., Kung, H.F., Gardier, A.M., Drarovsky, A., David, D.J., Deck, S.G., Hen, R. and Leonardo, E.D. (2010) 5-HT1A Autoreceptor Levels Determine Vulnerability to Stress and Response to Antidepressants. Neuron, 65, 40-51. http://dx.doi.org/10.1016/j.neuron.2009.12.003 [3] Berumen, L.C., Rodríquez, A., Miledi, R. and García-Alcour, G. (2012) Serotonin Receptors in Hippocampus. Scientific World Journal, 2012, Article ID: 823493. [4] Xu, T. and Pandey, S.C. (2000) Cellular Localization of Serotonin2A (5-HT2A) Receptors in Rat Brain. Brain Research Bulletin, 51,499-505. http://dx.doi.org/10.1016/S0361-9230(99)00278-6 [5] Bombardi, C. and Di Giovanni, G. (2013) Functional Anatomy of 5-HT2A Receptors in the Amygdale and Hippocampal Complex: Relevance to Memory Functions. Experimental Brain Research, 230, 427-439. http://dx.doi.org/10.1007/s00221-013-3512-6 [6] Sur, C., Betz, H. and Schloss, P. (1996) Immunocytochemical Detection of the Serotonin Transporter in Rat Brain. Neuroscience, 73, 217-231. http://dx.doi.org/10.1016/0306-4522(96)00030-9 [7] Tao-Cheng, J.H. and Zhou, F.C. (1999) Differential Polarization of Serotonin Transporters in Axons versus Soma-Dendrites: An Immunogold Electron Microscope Study. Neuroscience, 94, 821-830. http://dx.doi.org/10.1016/S0306-4522(99)00373-5 [8] Blakely, R.D., De Felice, L.J. and Hartzell, H.C. (1994) Physiology of Norepinephrine and Serotonin Receptors. The Journal of Experimental Biology, 196, 1263-281. [9] Breuer, M.E., Groenink, L., Oosting, R.S., Buerger. E., Korte, M., Ferger, B. and Olivier, B. (2009) Antidepressant Effects of Pramipexole, a DopamineD3/D2 Receptor Agonist, and 7-OH-DPAT, a Dopamine D3 Receptor Agonist, in M. R. Islam et al. 135 Olfactory Bulbectomized Rats. European Journal of Pharmacology, 616, 134-140. http://dx.doi.org/10.1016/j.ejphar.2009.06.029 [10] Hsuchou, H., Ho, Y.J., Shui, H.A., Tai, M.Y., Chen, K.H. and Tsai, Y.F. (2002) Effects of Incisor-Cutting on Muricidal Behavior Induced by Olfactory Bulbectomy in Rats. Physiology & Behavior, 76, 669-675. http://dx.doi.org/10.1016/S0031-9384(02)00807-7 [11] Hozumi, S., Nakagawasai, O., Tan-No, K., Niijima, F., Murata, A., Arai, Y., Yasuhara, H. and Tadano, T. (2003) Characteristics of Changes in Cholinergic Function and Impairment of Learning-Memory Related Behavior Induced by Olfactory Bulbectomy. Behavioral Brain Research, 138, 9-15. http://dx.doi.org/10.1016/S0166-4328(02)00183-3 [12] Aleksandrova, I.Y., Kuvichkin, V.V., Kashparov, I.A., Medvinskaya, N.I., Nesterova, I.V., Lunin, S.M., Samokhin, A.N. and Bobkova, N.V. (2004) Increased Level of β-Amyloid in the Brain of Bulbectomized Mice. Biochemistry (Moscow), 69, 176-180. http://dx.doi.org/10.1023/B:BIRY.0000018948.04559.ab [13] Han, F., Shioda, N., Moriguchi, S., Yamamoto, Y., Raie, A.A., Yamaguchi, Y., Hino, M. and Fukunaga, K. (2008) Spiro[imidazo[1,2-a]pyridine-3,2-indan]-2(3H)-one (ZSET1446/ST101) Treatment Rescues Olfactory BulbectomyInduced Memory Impairment by Activation of Ca2+/Calmodulin Kinase II and Preotin Kinase C in Mouse Hippocampus. The Journal of Pharmacology and Experimental Therapeutics, 326, 127-134. http://dx.doi.org/10.1124/jpet.108.137471 [14] Shioda, N., Yamamoto, Y., Han, F., Moriguchi, S., Yamaguchi, Y., Hino, M. and Fukunaga, K. (2010) A Novel Cognitive Enhancer, ASET1446/ST101, Promotes Hippocampal Neurogenesis and Ameliorates Depressive Behavior in Olfactory Bulbectomized Mice. Journal of Pharmacology and Experimental Therapeutics, 333, 43-50. http://dx.doi.org/10.1124/jpet.109.163535 [15] Neckers, L.M., Zarrow, M.X., Myers, M.M. and Denenberg, V.H. (1975) Influence of Olfactory Bulbectomy and the Serotonergic System upon Intermale Aggression and Maternal Behavior in the Mouse. Pharmacology Biochemistry and Behavior, 3, 545-550. http://dx.doi.org/10.1016/0091-3057(75)90170-7 [16] Watanabe, A., Tohyama, Y., Nguyen, K.Q., Hasegawa, S., Debonnel, G. and Diksic, M. (2003) Regional Brain Serotonin Synthesis Is Increased in the Olfactory Rat Model of Depression: An Autoradiographic Study. Journal of Neurochemistry, 85, 469-475. http://dx.doi.org/10.1046/j.1471-4159.2003.01702.x [17] Cross, A.J., Crow, T.J., Ferrier, I.N., Johnson, J.A., Bloom, S.R. and Corsellis, J.A. (1984) Serotonin Receptor Changes in Dementia of the Alzhemier Type. Journal of Neurochemistry, 43, 1574-1581. http://dx.doi.org/10.1111/j.1471-4159.1984.tb06081.x [18] Mann, D.M. and Yates, P.O. (1983) Serotonin Nerve Cells in Alzheimer’s Disease. Journal of Neurology, Neurosurgery & Psychiatry, 46, 96-98. http://dx.doi.org/10.1136/jnnp.46.1.96 [19] Lai, M.K., Tsang, S.W., Esiri, M.M., Francis, P.T., Wong, P.T. and Chen, C.P. (2011) Differential Involvement of Hippocampal Serotonin1A Receptors and Re-Uptake Sites in Non-Cognitive Behaviors of Alzheimer’s Disease. Psychopharmacology, 213, 431-439. http://dx.doi.org/10.1007/s00213-010-1936-2 [20] Wilkinson, D.G., Francis, P.T., Schwam, E. and Payne-Parrish, J. (2004) Cholinesterase Inhibitors Used in the Treatment of Alzheimer’s Disease: The Relationship between Pharmacological Effects and Clinical Efficacy. Drugs & Aging, 21, 453-478. http://dx.doi.org/10.2165/00002512-200421070-00004 [21] Mowla, A., Mosavinasab, M., Haghshenas, H. and Borhani Haghighi, A. (2007) Does Serotonin Augmentation Have Any Effect on Cognition and Activities of Daily Living in Alzheimer’s Dementia? A Double-Blind, Placebo-Controlled Clinical Trial. Journal of Clinical Psychopharmacology, 27, 484-487. [22] McDermott, C.L. and Gray, S.L. (2012) Cholinesterase Inhibitor Adjunctive Therapy for Cognitive Impairment and Depressive Symptoms in Older Adults with Depression. Annals of Pharmacotherapy, 46, 599-605. http://dx.doi.org/10.1345/aph.1Q445 [23] Spalletta, G., Gianni, W., Giubilei, F., Casini, A.R., Sancesario, G., Caltaqirone, C. and Cravello, L. (2013) Rivastigmine Patch Ameliorates Depression in Mild AD: Preliminary Evidence from a 6-Month Open-Label Observational Study. Alzheimer Disease & Association Disorder, 27, 289-291. http://dx.doi.org/10.1097/WAD.0b013e318260ab0a [24] Moriguchi, S., Tagashira, H., Sasaki, Y., Yeh, J.Z., Sakagami, H., Narahashi, T. and Fukunaga, K. (2014) CaMKII Activity Is Essential for Improvement of Memory-Related Behaviors by Chronic Rivastigmine Treatment. Journal of Neurochemistry, 128, 927-937. http://dx.doi.org/10.1111/jnc.12510 [25] Islam, M.R., Moriguchi, S., Tagashira, H. and Fukunaga, K. (2014) Rivastigmine Improves Hippocampal Neurogenesis and Depression-Like Behaviors via 5-HT1A Receptor Stimulation in Olfactory Bulbectomized Mice. Neuroscience, 272, 116-130. http://dx.doi.org/10.1016/j.neuroscience.2014.04.046 [26] Singer, S., Rossi, S., Verzosa, S., Hashim, A., Konow, R., Cooper, T., Serchen, H. and Lajtha, A. (2004) Nicotine-Induced Changes in Neurotransmitter Levels in Brain Areas Associated with Cognitive Function. Neurochemical Research, 29, 1779-1792. http://dx.doi.org/10.1023/B:NERE.0000035814.45494.15 M. R. Islam et al. 136 [27] Jarosik, J., Legutko, B., Unsicker, K. and von Bohlen Und Halbach, O. (2007) Antidepressant-Mediated Reversal of Abnormal Behavior and Neurodegenaration in Mice Following Olfactory Bulbectomy. Experimental Neurology, 204, 20-28. [28] Westlind, A., Grynfarb, M., Hedlund, B., Bartfai, T. and Fuxe, K. (1981) Muscarinic Supersentivity Induced by Septal Lesion or Chronic Atropine Treatment. Brain Research, 225, 131-141. http://dx.doi.org/10.1016/0006-8993(81)90323-1 [29] Chen, Y., Shohami, E., Bass, R. and Weinstock, M. (1998) Cerebro-Protective Effects of ENA-713, a Novel Acetylcholinesterase Inhibitor, in Closed Head Injury in the Rat. Brain Research, 784, 18-24. http://dx.doi.org/10.1016/S0006-8993(97)00982-7 [30] Rao, T., Correa, L.D., Reid, R.T. and Lioyd, G.K. (1996) Evaluation of Anti-Nociceptive Effects of Neuronal Nicotinic Acetylcholine Receptor (nAChR) Ligands in the Rat Tail-Flick Assay. Neuropharmacology, 35, 393-405. http://dx.doi.org/10.1016/0028-3908(96)00013-5 [31] Kenny, P.J., File, S.E. and Neal, M.J. (2000) Evidence for a Complex Influence of Nicotinic Acetylcholine Receptors on Hippocampal Serotonin Release. Journal of Neurochemistry, 75, 2409-2414. http://dx.doi.org/10.1046/j.1471-4159.2000.0752409.x [32] De la Garza II, R. and Yoon, J.H. (2011) Evaluation of the Effects of Rivastigmine on Cigarette Smoking by Methamphetamine-Dependent Volunteers. Progress in Neuro-Psychopharmacology & Biological Psychiatry, 35, 1827-1830. [33] Kenny, P.J., Cheeta, S. and File, S.E. (2000) The Anxiogenic Effects of Nicotine in the Dorsal Hippocampus Are Mediated by 5-HT1A and Not Muscarinic M1 Receptors. Neuropharmacology, 39, 300-307. http://dx.doi.org/10.1016/S0028-3908(99)00114-8 [34] Reid, R.T. and Sabbagh, M.N. (2008) Effects of Cholinesterase Inhibitors on Rat Nicotinic Receptors Levels in Vivo and in Vitro. Journal of Neural Transmission, 115, 1437-1444. http://dx.doi.org/10.1007/s00702-008-0107-7 [35] Nizri, E., Irony-Tur-Sinai, M., Faranesh, N., Lavon, I., Lavi, E., Weinstock, M. and Benner, T. (2008) Suppression of Neuroinflammation and Immunomodulation by the Acetylcholinesterase Inhibitor Rivastigmine. Journal of Neuroimmunology, 203, 12-22. http://dx.doi.org/10.1016/j.jneuroim.2008.06.018 [36] Sato, H., Skelin, I. and Diksic, M. (2010) Chronic Busiprone Treatment Decreases 5-HT1B Receptor Densities and the Serotonin Transporter but Increases the Density of 5-HT2A Receptors in the Bulbectomied Rat Model of Depression: An Autoradiographic Study. Brain Research, 1345, 28-44. http://dx.doi.org/10.1016/j.brainres.2010.05.054 [37] Kato, K., Awasaki, T. and Ito, K. (2009) Neuronal Programmed Cell Death Induces Glial Cell Division in the Adult Drosophila Brain. Development, 136, 51-59. http://dx.doi.org/10.1242/dev.023366